Origins of the MEG signal
Brain structure
The brain is composed of around 80 billion neurons. On the macroscopic level the brain is arranged in functional areas. The different cortical lobes and areas of the brain do specific tasks like motor function, somatosensory function, visual and auditory processing and many more. Then there are the subcortical structures that act as signal intermediaries, relay stations, fine tuning and so on. The microscopic structure of the brain is quite uniform along the cortex, with some change in organization when entering the hippocampal areas.
The microscopical structure influences the magnetic field outside of the head as the sum electrical field produces the magnetic field that is visible to the MEG. It is generally agreed that the largest contributing neural generator of the MEG signal are the cortical pyramidal neurons. These neurons transverse the six cortical layers in a distinct orientation perpendicular to the cortical surface. Other neuronal structures transverse the cortical layers in somewhat random orientations, so the net electrical current is zero.
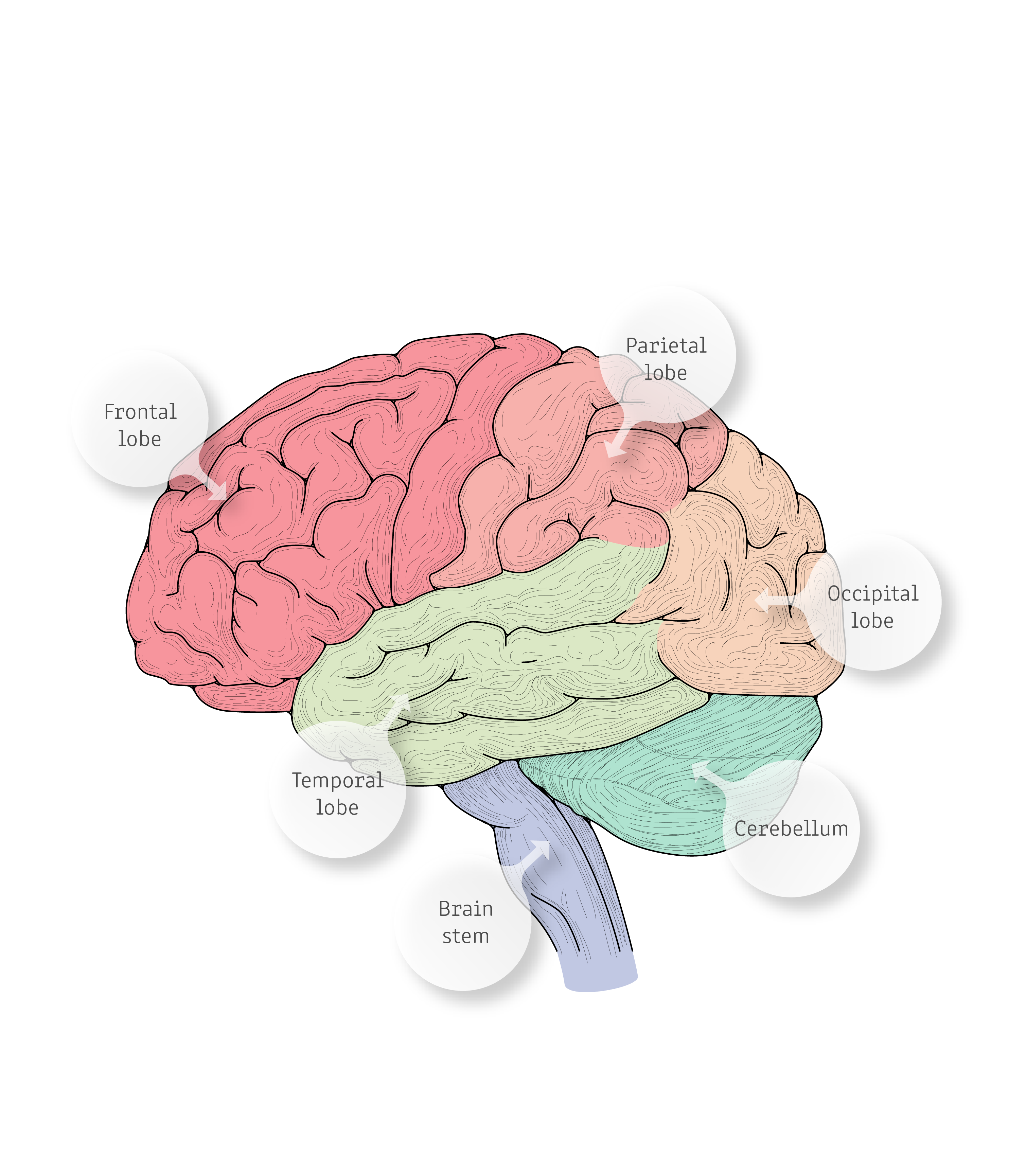
Image 9. Brain anatomy with cortical lobes, cerebellum and brainstem.
Neurons and different types of currents in the brain
The pyramidal neurons populate the whole of the cortex. A total of around 50 000 pyramidal neurons are required to elicit a recordable neural current. When an electrical signal is transmitted along a neuron, electrical charges are separated along very short distances on the corresponding cell membranes. These charges form a sort of temporary "battery", with positive polarity at the side where the current is leaving and with negative polarity where the current is returning. These "batteries" are called primary currents. They directly reflect the activity of the corresponding neurons. The primary currents are embedded in a conductive medium, i.e., the brain tissue and brain liquor. Therefore, a current is induced which flows through these media, as well as the skull and scalp, called secondary or volume currents. These volume currents also reach the scalp surface and cause voltage differences at the scalp that can be picked up by EEG electrodes. Both primary and volume currents produce magnetic fields, which sum up and can be measured by pick-up coils above the head using MEG. Possible contributors to the measurable signals are:
(1) action potentials along the axons connecting neurons
With action potentials, two opposing currents occur in vicinity of each other, thus cancelling each other. Also, action potentials last only a few milliseconds, making it unlikely to record larger numbers of simultaneous action potentials summing up to a measurable signal at a distance.
(2) currents through the synaptic clefts connecting axons with neurons/dendrites
Synapses are very small and the current flow relatively slow. Furthermore, they can be located nearly randomly around a neuron and its dendrites, so that the contributions of different synapses are likely to cancel each other out.
(3) Currents along dendrites from synapses to the soma of neurons.
The current flow along post-synaptic dendrites is dipolar, and at least the apical dendrites in cortical pyramidal cells are generally parallel organized. They are typically active for about 10ms after synaptic input. For these reasons, the apical dendrites of the cortex are assumed to contribute strongest to the measurable EEG and MEG signals.
Because this activity directly reflects processing of specific neurons, EEG and MEG are the most direct correlate of on-line brain processing obtainable non-invasively. However, one dendrite would be far too weak to produce a measurable signal, instead tens of thousands are required to be active synchronously. Consequently, EEG/MEG are only sensitive to coherent simultaneous activity of many neurons. For modelling purposes, it is important to note that many weak neighboring dipolar sources can be represented as a dipole, which can be interpreted as a piece of active cortex.
Since the primary currents are oriented perpendicular to the cortical surface heading inwards, with the right-hand rule we can see that the accompanying magnetic field must be a rotating field with its rotational axis tangential to the cortical surface. If we investigate this further, we can see that radial electrical current on the top of the brain gyri and on the bottom of the sulci, aka currents pointing to the center of the brain, produce little to no noticeable magnetic field outside the skull. Inversely, the walls of the gyri produce a magnetic field that radiates outward from the head and is readily noticeable by the MEG sensors. This idea is conveyed in the image presented below (Primary current in blue and brown, induced magentic field in green).
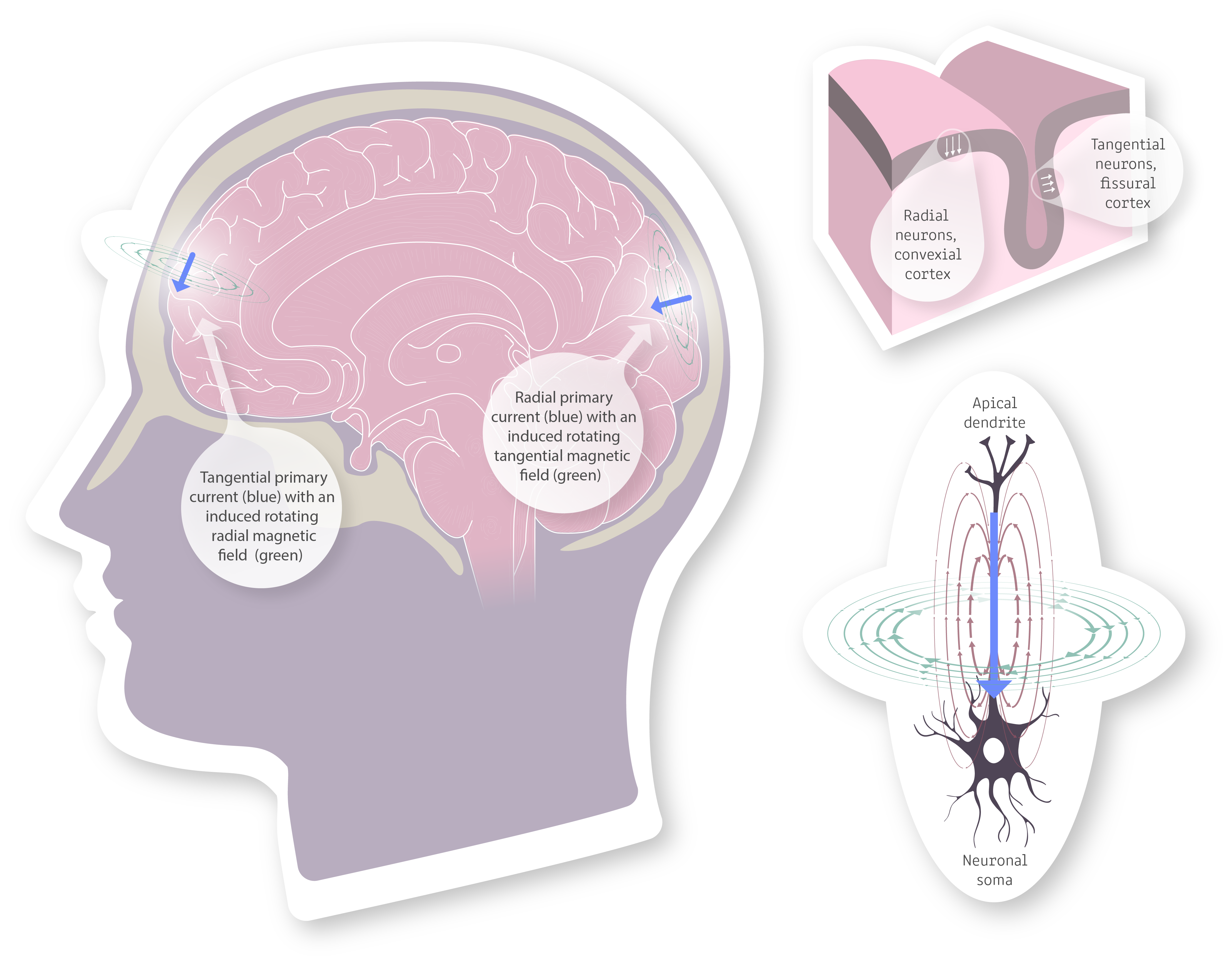
Image 10. On the left we have an illustration of primary currents (blue) and the magnetic fields (green) they induce. We can see that the tangential proimary currents are the primary source of cortical extra cranial magnetic fields, as the radial primary current induces a tangential magnetic field that does not project outward from the cranium. Top right represens a slab of cortical tissue with arrows (white) showing the orientation of primary currents generated by neuronal axons. The fissural cortex is thus the main area with tangentially oriented neurons and gives rise to the bulk of the magnetic field the MEG can observe. Bottom right zooms in on a single cortical pyramidal neuron and looks at the primary current (blue), the electrical field (violet-brown) and the magnetic field (green) generated by the neuron.
Influence of cranial tissues
The cranial tissues, brains, CNS liquor, blood, meninges, skull, and skin, all contribute to the conductance and electrical properties of the head. This poses a problem for accurate localization of the EEG data since the electrical currents traverse the tissues at different rates and the accurate electrophysiological modeling of all the different tissues is extremely difficult. On the other hand, neural magnetic fields are virtually unaffected by the cranial tissues since the tissues have near negligeable magnetic permeability.
When localizing or modeling the three-dimensional magnetic topography of MEG signals, 3 concentric spheres can be used as a substitute for the actual brain anatomy, and it still yields quite accurate results. The localization can be refined further with individual anatomical brain MRIs. The MRIs are then used to generate the boundary element model (BEM) of the brain which can be used to accurately project MEG signal activation onto the individual brain anatomy.
Noise sources affecting the extracranial magnetic fields
The noise sources can be divided into external and internal magnetic noise. The internal magnetic noise arises from the subject and is of non-neural origin. Then there are external noise sources that arise from outside the body
Internal magnetic fields
- Eye movement – saccades, blinks, retinal signals
- Heart beat – periodic magnetic pulse
- Breathing – small drifts in the data
- Muscle tension – especially in the head and neck
- Muscle movements – head, arms
- Magnetized implants etc.
External magnetic fields
- Traffic
- Electric lines
- Motors and devices
- Doors, elevators, other moving metal
- Vibrations of the building
- Earth