General introduction
What is MEG?
Magnetoencephalography (MEG) is a brain imaging modality that maps brain activity by sampling tiny magnetic fields produced by electrical currents occurring naturally in the brain. MEG measures the brain activity in the scale of neuronal systems and networks. It is closely related to the older imaging modality electroencephalography (EEG), which measures brain activity by measuring electrical currents from the scalp.
The strengths of MEG are its great temporal accuracy, good spatial accuracy, and non-invasiveness. On the temporal side, MEG is unrivaled since it can accurately measure brain activity within a range of a few milliseconds. Optimal and interference free measurements yield the possibility of good spatial accuracy with MEG. The problem with spatial accuracy is the ill-posed inverse problem. As the brain is composed of around 86 billion neurons and around 15 billion cortical neurons, the MEG with its 300 sensors can’t reliably decode brain activity as such. That said, the advances in computing and modern approaches to the problem allow the MEG to map brain activity with relatively good spatial accuracy. Many of the approaches utilize a priori information, aka. additional information through which the sensor data can be decoded to yield a single solution to the inverse problem. Finally, the MEG is excellent in its non-invasiveness. Compared to EEG, MEG can record just with the subject sitting in the measurement seat with their head in the MEG helmet. Often additional electrodes and coils are attached to the subject’s head, but this is not explicitly necessary. Compared to say magnetic resonance imaging (MRI), in MEG the subject is seated comfortably and there is little to no noise from the equipment.
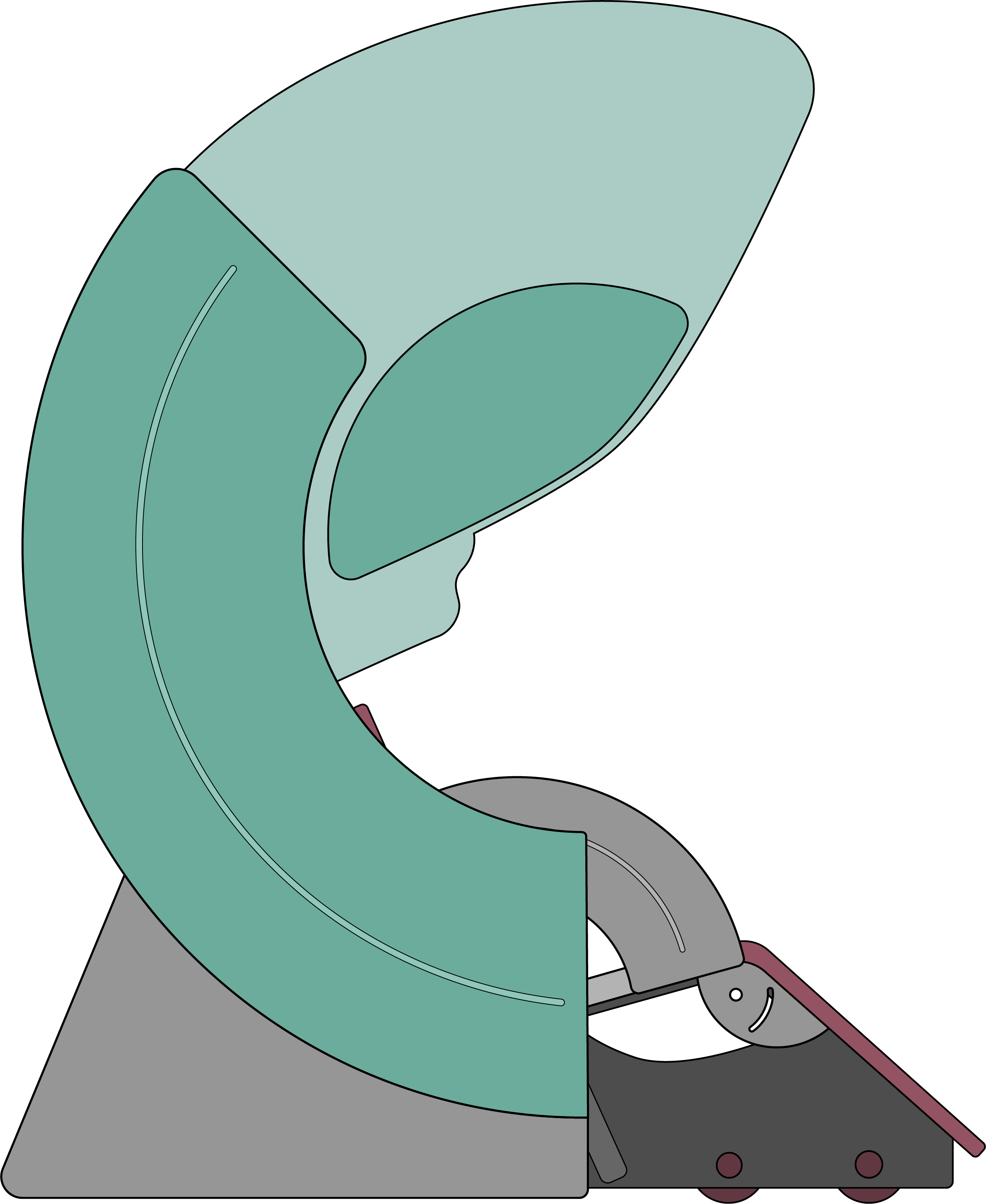
Image 1. An illustration of a typical meg scanner.
Some of the weaknesses of MEG are its cost, somewhat limited clinical uses, and sensitivity to interference. A modern full head MEG system costs upwards of 1-2 million USD, and the ones that rely on SQUID-sensors have maintenance costs and might require helium refills. MEG scanners are quite sensitive to external magnetic fields as a side product of their operating mechanism. To combat the effects of external magnetic fields the MEG scanners are housed in a shielded rooms and preferably separated from the foundation of the rest of the building. There are also hardware and software shielding mechanisms that can be used to attenuate the magnetic interference. One of the limiting factors of wide scale adoption of MEG scanners is their relatively limited clinical use. MEG scanners have been used in epilepsy diagnostics and both tumor and epilepsy surgery as a presurgical method for identifying and mapping brain areas of interest.
Many of the MEGs weaknesses have been reduced with state-of-the-art technology in both hardware and software advancements in the last 2 decades. Also, there are numerous emerging neurophysiological uses for MEG, and other functional neuroimaging methods, being investigated and validated around the world.
Comparing MEG to other imaging methods
There are numerous brain imaging methods that have their strengths and weaknesses. When comparing MEG to other methods we can look at a few different aspects. The most important things to look at are the spatial and temporal acuity of the method. Other important points are subject comfort and invasiveness of the method. MEG is concidered a functional neuronal imaging method where some other imaging methods are more suited for anatomical imaging of the brain.
fMRI vs MEG
Functional magnetic resonance imaging or functional MRI (fMRI) measures brain activity by detecting changes associated with blood flow within the brain. This technique is predominantly used to localize brain functions prior to surgery. The difference between these two techniques predominantly lies in that fMRI measures blood flow relying on the fact that cerebral blood flow and neuronal activation are coupled. MEG directly measures brain activity through the magnetic field the neuronal activation produces. Consequently, MEG has a much higher temporal resolution than fMRI, allowing the timing of brain activity to be much more precisely measured.
Image 2. An illustration of the origins of a few imaging modalities.
EEG vs MEG
Like MEG, EEG measures and records electrical activity in the brain. However, unlike EEG, MEG can tell where in the brain this functional activity originates. This is because the human skull and the tissue surrounding the brain distort the electrical signals an EEG picks up, but affects magnetic fields produced by those electric signals much less and in a measurable way that can be accounted for.
EEG and MEG are great tools to use in conjunction with one another as the information they offer complement one another.
iEEG vs MEG
Intracranial electroencephalography is a technique that measures and records electrical brain activity just as scalp EEG does. However, iEEG requires electrodes to be implanted in the brain. This allows it to be much more accurate with respect to the location and timing of electrical activity in the brain. However, this method requires brain surgery. And because of this, iEEG cannot be used for all brain areas due to difficulty of access to all parts of the brain. MEG allows for accurate measurement of the location and timing of electrical activity in the brain, in a quick and easy non-invasive scan.
SPECT & PET vs MEG
A single photon emission computed tomography (SPECT) scan and a positron emission tomography (PET) are imaging modalities that show how blood flows to tissues and organs. Both are nuclear imaging scans that integrates computed tomography (CT) and a radioactive tracer. The tracer is what allows doctors to see how blood flows to tissues and organs. Before the scan, a tracer is injected into the bloodstream. This imaging technique is used to form a 3D image of the brain. MEG is completely non-invasive and does not require any tracer, radioactive or otherwise, to be injected.
CT vs MEG
A computerized tomography (CT) scan combines a series of X-ray images taken from different angles around your body and uses computer processing to create cross-sectional images of the bones, blood vessels and soft tissues inside your body. A CT scan is a standard imaging modality used to assess the brain. However, because it only creates a structural image of the brain, it is not an imaging technique that can determine how the brain is functioning. In addition, CT scans use ionizing radiation which has given rise to concern about radiation exposure in patients who have had many scans. MEG is completely non-invasive and does not require the use of radiation to detect brain function.
MRI vs MEG
Magnetic resonance imaging (MRI) is a medical imaging technique used to form pictures of the anatomy and the physiological processes of the body. MRI scanners use strong magnetic fields, magnetic field gradients, and radio waves to generate images of the organs in the body. Because of its use of magnets to image the body, some patients with certain types of implants are not able to have an MRI scan. However, like CT, MRIs creates a structural image of the brain and therefore isn’t an imaging technique that can assess how the brain is functioning.
TMS-EEG vs MEG
Transcranial magnetic stimulation (TMS) optionally with EEG is an emerging method for studying the brain’s cortical network properties. TMS itself is older and has been used in e.g. examining cortical excitability and pseud-lesion studies. TMS activates the cortex via a magnetic pulse that induces a current in the desired cortical region. The method can be considered invasive. TMS(-EEG) is quite spatially accurate on the apical cortical areas. MEG is temporally slightly more accurate than TMS-EEG. MEG is non-invasive and more comfortable for the subject. MEG cannot affect the cortical function so studies like the TMS pseudo-lesion studies cannot be conducted with MEG.
Applications
Research applications
MEG as a research tool is quite versatile in giving insight into neurobehavioral and cognitive research questions. Like EEG, MEG can decode a variety of different metrics that can be used in studying different aspects of cognition. Popular research topics are visual, auditory and language processing, memory function. It has also given unique insight on the time-resolved processes of brain functions and network integration including resting-state dynamics.
The oldest research methods used in MEG research are the evoked and induced responses to a given stimuli. Looking at the activation locations and patterns we can get information of the brain’s functional organization and the temporal hierarchy of activation. Further, with the help of modern signal analysis and Fourier theory, we can decode MEG data to reveal the power of the ongoing oscillatory activity in relation to a timed stimulus or then the resting brain. These can reveal things like local synchrony or desynchrony and give insight to the activity of brain networks.
Nowadays MEG research still utilizes these older methods, but newer methods and analyses have gained traction in the scientific community. One such direction is ‘single-trial analyses’, where the variability of brain responses and behavioral changes are taken into consideration. Another newer direction are connectivity studies in which, e.g., the similarity between rhythmic activation of the brain is decoded and the connectivity between brain regions is analyzed.
The older and the newer methods work often in tandem, complimenting each other, to give astounding insights into the workings of the brain. The advancements in methods, technology, and concepts, combined with MEG’s inherent advantages, create an exciting and useful tool in the hands of cognitive neuroscientists.
MEG has strong value in revealing the dynamics of brain activity in sensory perception, cognition, and behavior: it has provided unique insight on the time-resolved processes of brain functions and network integration including resting-state dynamics.
Clinical applications
In 2017 it was calculated that there are about 200 whole-head MEG scanners in the world. Many of them reside in clinical environments like hospitals. Still, the clinical applications of the MEG remain scarce. The most well-known applications are epilepsy diagnostics and pre-neurosurgical workups to determine localization of functional areas in the brain.
With the amount of functional neuroimaging research data increasing, there are new indications for clinical MEG applications in the horizon. The research areas now showing great potential are the use of neuroimaging in the assessment/diagnosis of neurological and psychiatric syndromes, developmental disorders, and the integrity of cortical brain networks after stroke.